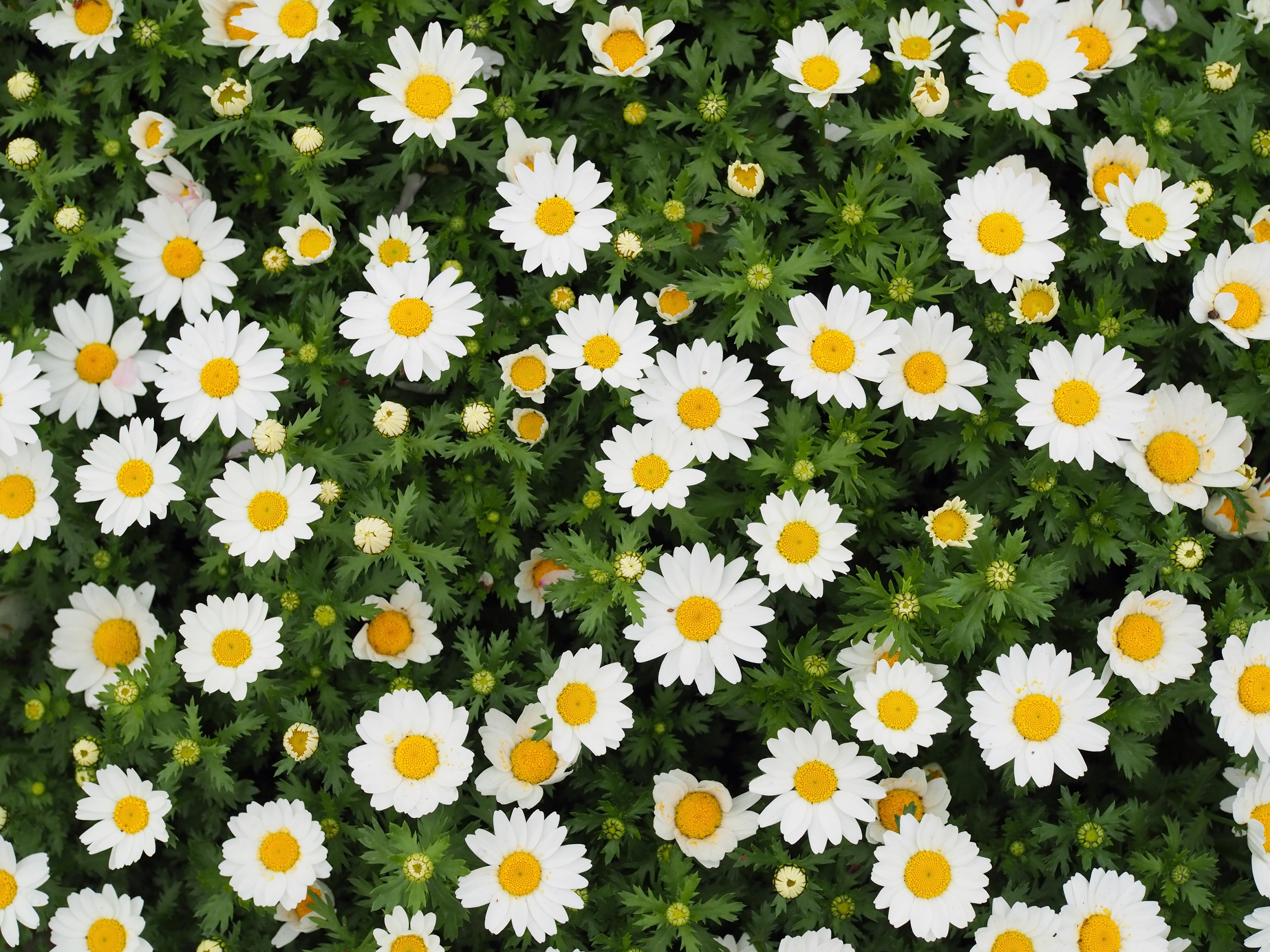
With your help, everything can change
Whether it’s writing to your MP, planting bee-friendly flowers or giving £5 a month – take action today and join the good food revolution!
Whether it’s writing to your MP, planting bee-friendly flowers or giving £5 a month – take action today and join the good food revolution!